Virus Mechanics Project
BACKGROUND
PROJECT INFO
Public awareness of virus structure and function is a crucial aspect of health education, supporting vaccine acceptance and enabling informed decisions about the role of vaccination and antivirals in the control of disease. This work contributes to public health educational materials and activities to combat vaccine hesitancy, identified by the World Health Organization as one of the top ten global health threats in 2019.
This project focuses on models as essential tools to help describe and explain scientific ideas, aiding in the understanding of phenomena. The aim was to develop a dynamic 3D model to communicate the mechanics of viral infection, which learners can manipulate as part of a ‘hands-on, minds-on’ approach for educational engagement.
The initial prototype focused on poliomyelitis (commonly known as polio) as a model system of virus anatomy and behaviour. Like many viruses, poliovirus undergoes a structural change when it interacts with a living cell. This change involves the movement of proteins on the virus shell (also known as the ‘capsid’), allowing the injection of genetic material to infect the living cell.
Year
2019 – 2020
Collaborators
Briony Thomas, Project Lead & Creative Direction
Kacper Dobras, Design Research & Fabrication
Keith Grehan, Scientific Advisor
Funder
University of Leeds
GALLERY
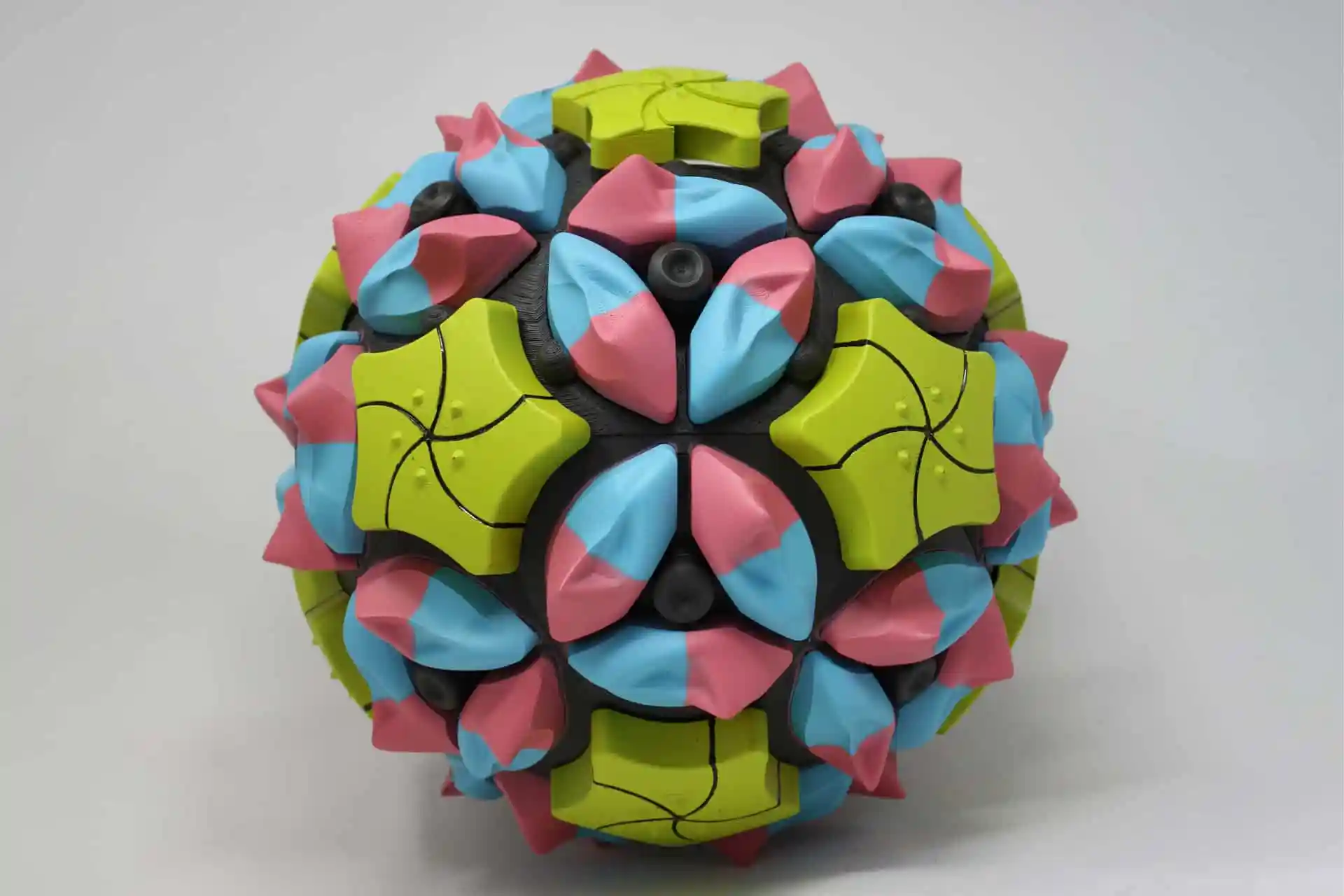
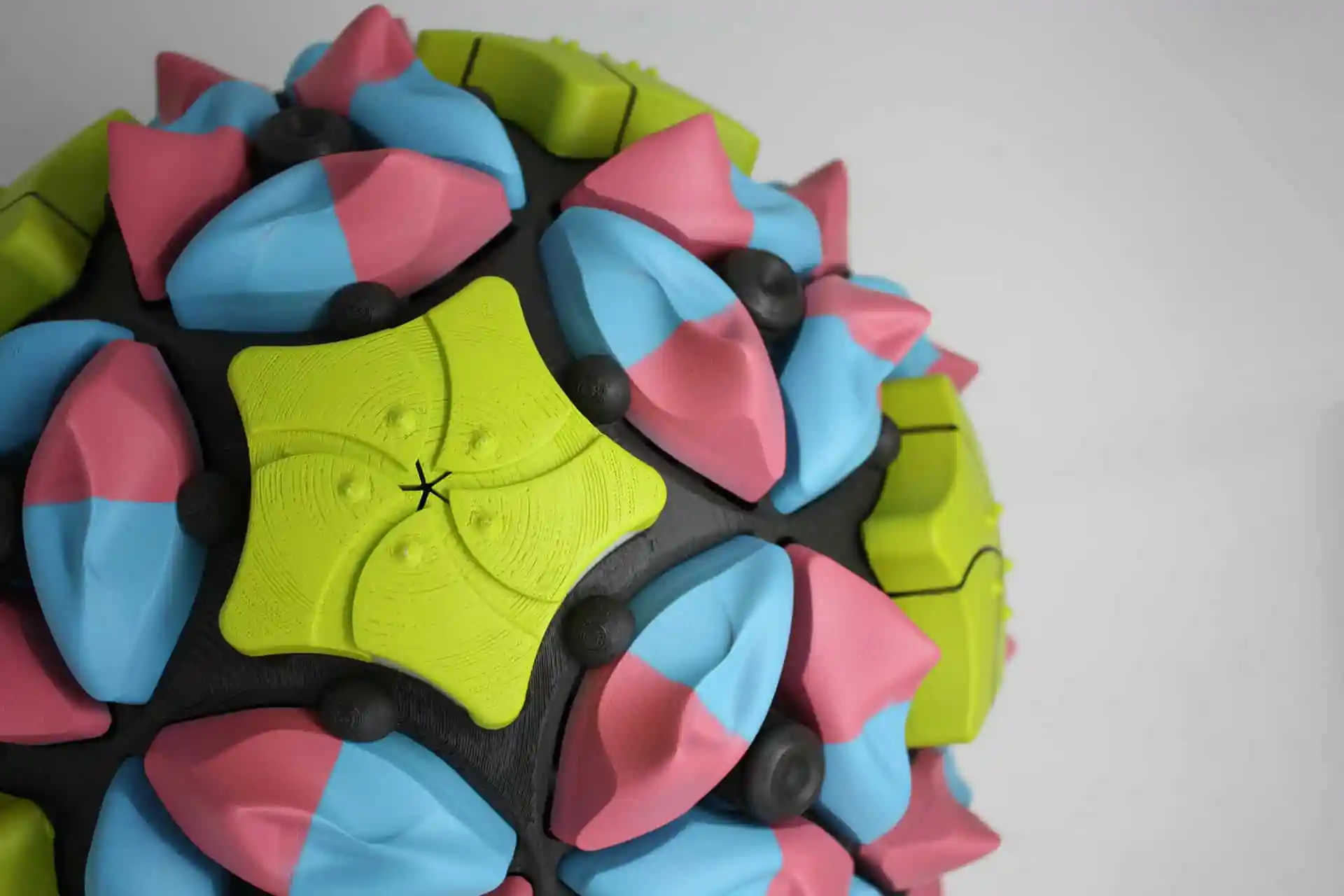
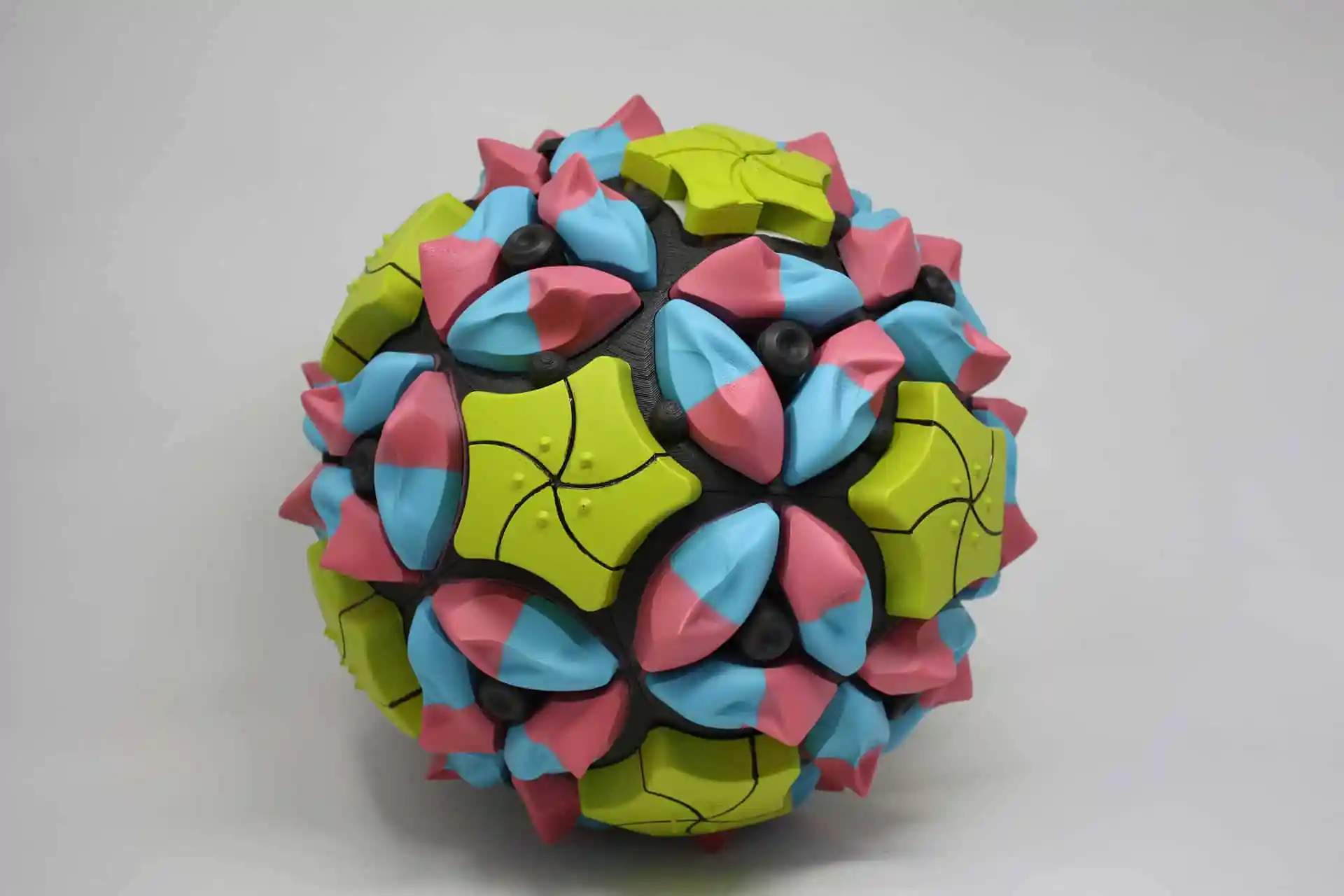
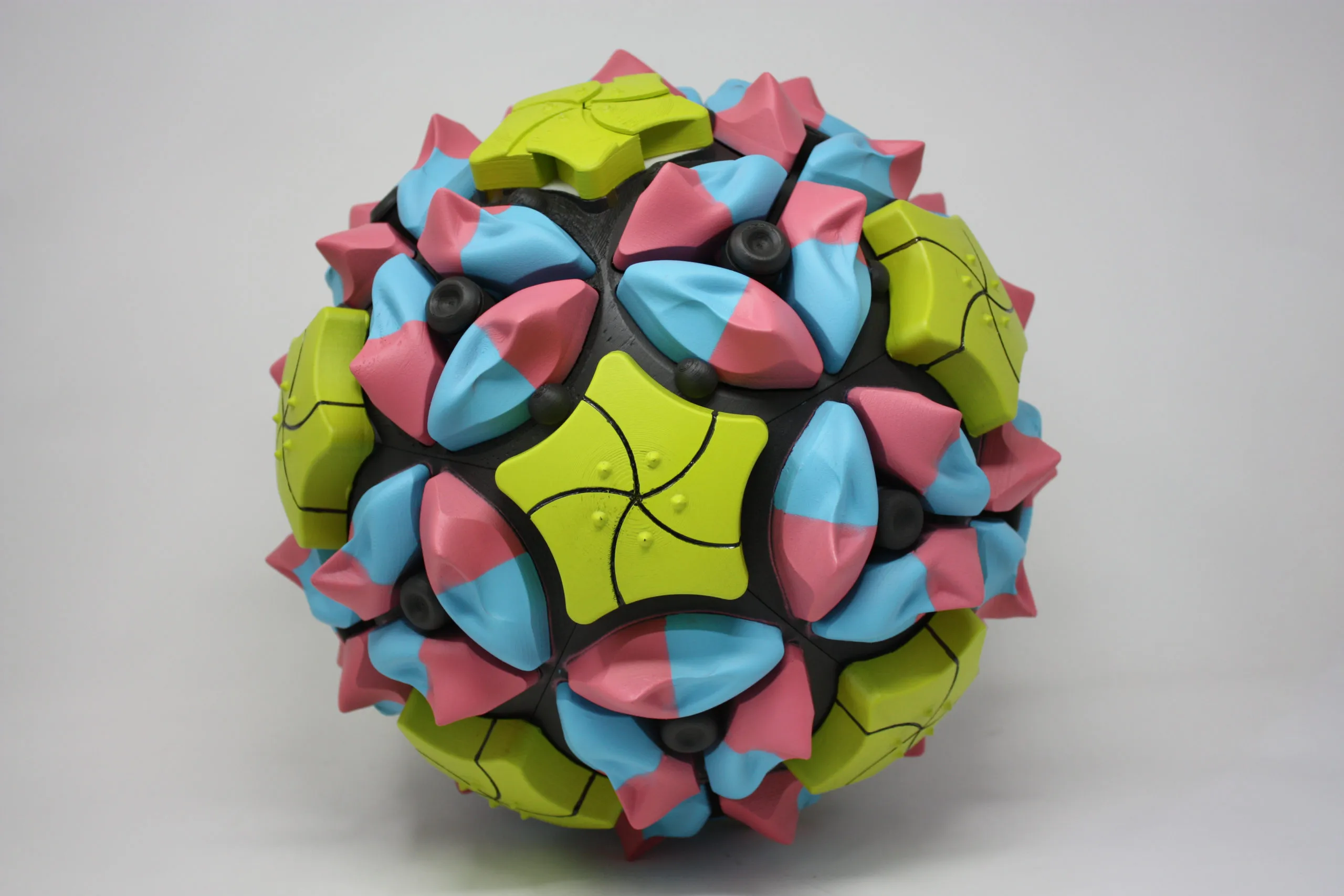
EXPLORE IN AR
DESIGN
The poliovirus capsid is a dynamic structure four repeating structural proteins — virus proteins 1–4 (VP1, VP2, VP3, and VP4). The capsid is composed of 60 copies of these proteins, arranged with icosahedral symmetry. The goal was to create a model that demonstrates the multicomponent viral capsid and the changes that occur at the early stage of infection. The geometric structure of the capsid played a crucial role in the description of the biological structure. It was key in enabling the representational modelling.
The topology of the poliovirus capsid is modelled below, showing the key elements of its biological structure. VP1, VP2 and VP3 form the surface of the capsid and VP4 is an internal protein that stabilises the capsid structure. VP1 forms a prominent star-shape at the fivefold axis of symmetry, known as the ‘mesa’. It is surrounded by a deep depression, known as the ‘canyon’. Another structural feature, known as the ‘propeller,’ is formed by VP2 and VP3 at the threefold axis of symmetry.
The majority of models present the virus fixed in one of two states, either before or after the conformational change. The challenge was to translate its microscopic mechanical features into a dynamic model that demonstrates the capsid transformation through this change, modelling ‘inactive’ and ‘active’ states of the virus.
This design enables changes to the topology of the capsid model as it transforms between states. In its inactive state, the shape of the mesa resembles a star. Transformation into an active state causes arms of the star to move, opening the centre of the mesa. The opening of the mesa allows the VP4 ‘claw’ to expand and become visible. Light shines through the mesa, visualising the transmission of genomic material to cause infection, as shown below.
PROCESS
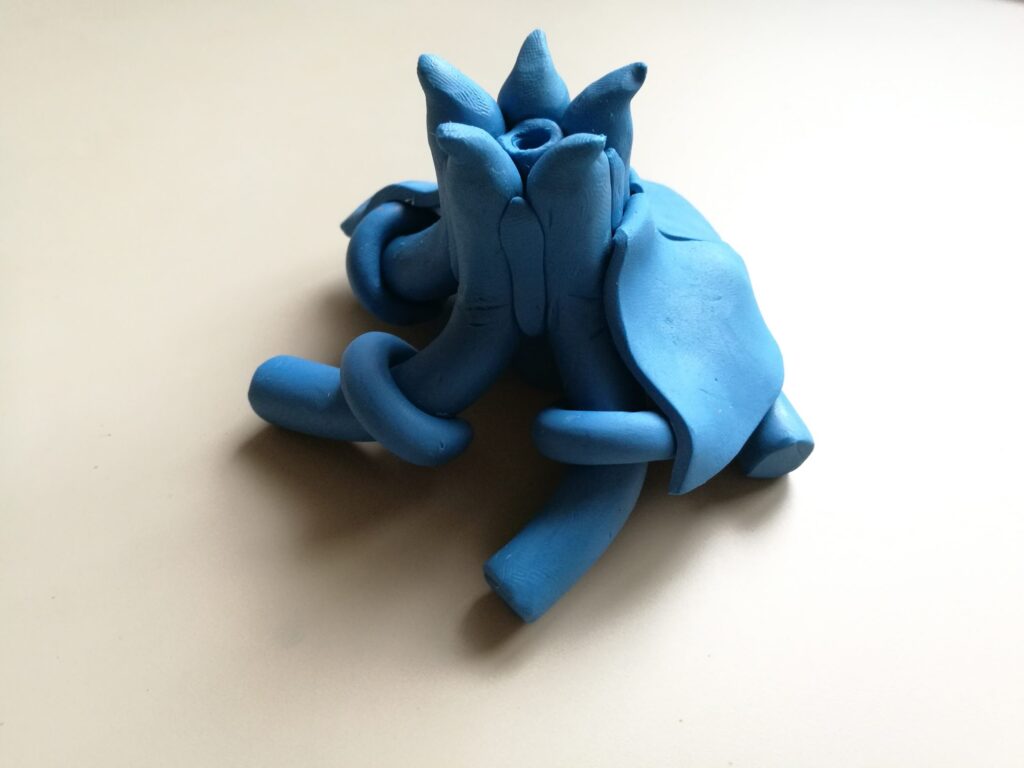
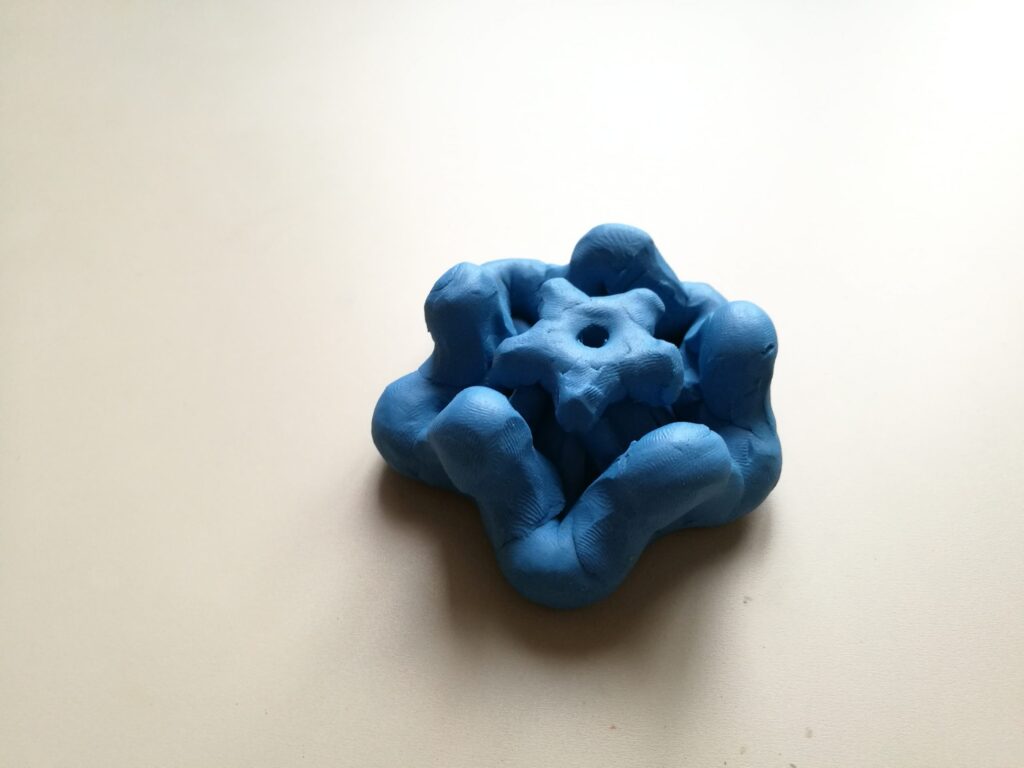
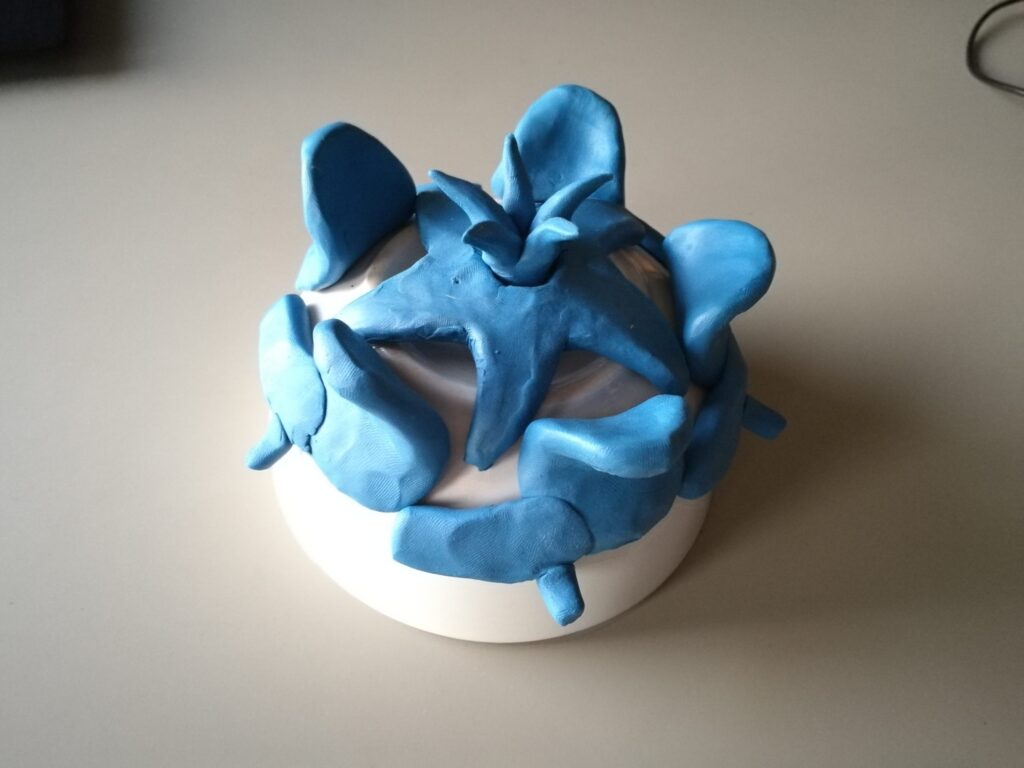
A testing prototype — comprising one star-shaped mesa region — was digitally fabricated to assess usability with potential stakeholders. The design of testing prototype is pictured below, both in CAD render and 3D printed form. The design of the dome-shaped testing prototype is based on a mechanical iris, similar to a camera lens aperture.
During the testing session, users engaged with exploratory design sketches and the testing prototype to understand the infection process. Mechanical aspects of the model were also explored through assembly and disassembly of the prototype by the group. This feedback provided valuable insights for revisions of the prototype mechanisms and the final iterations of the design.
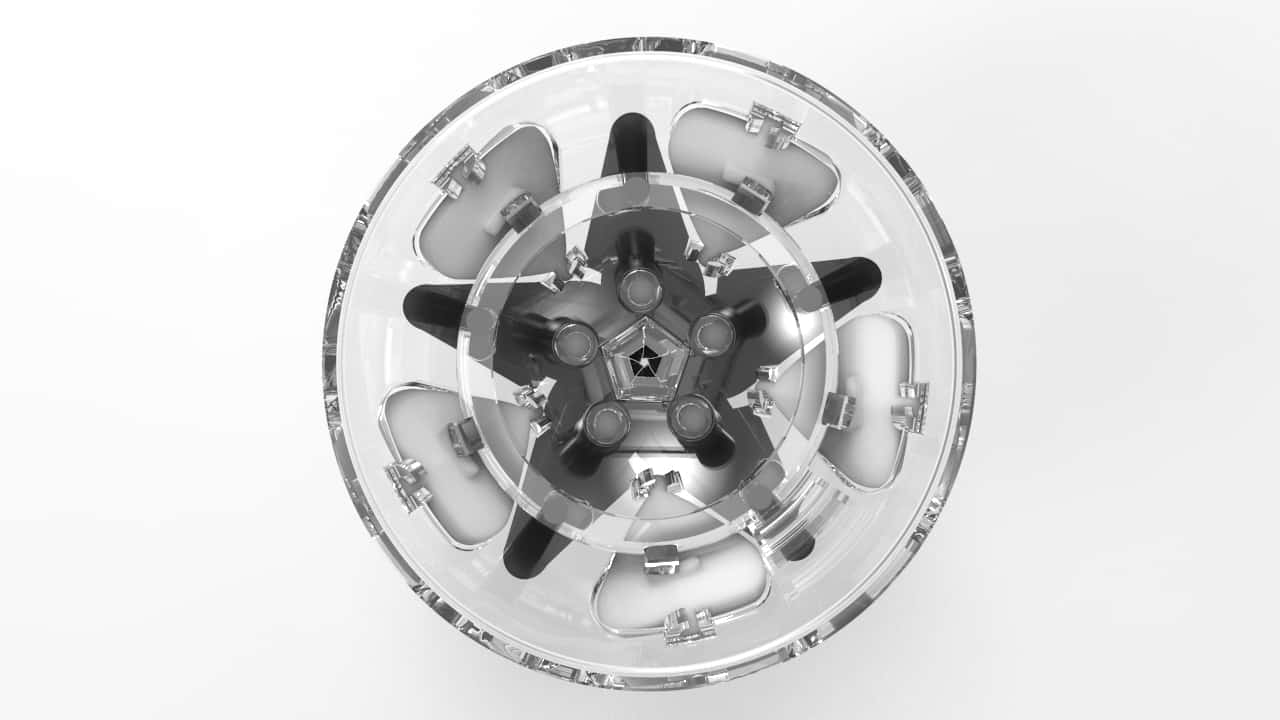
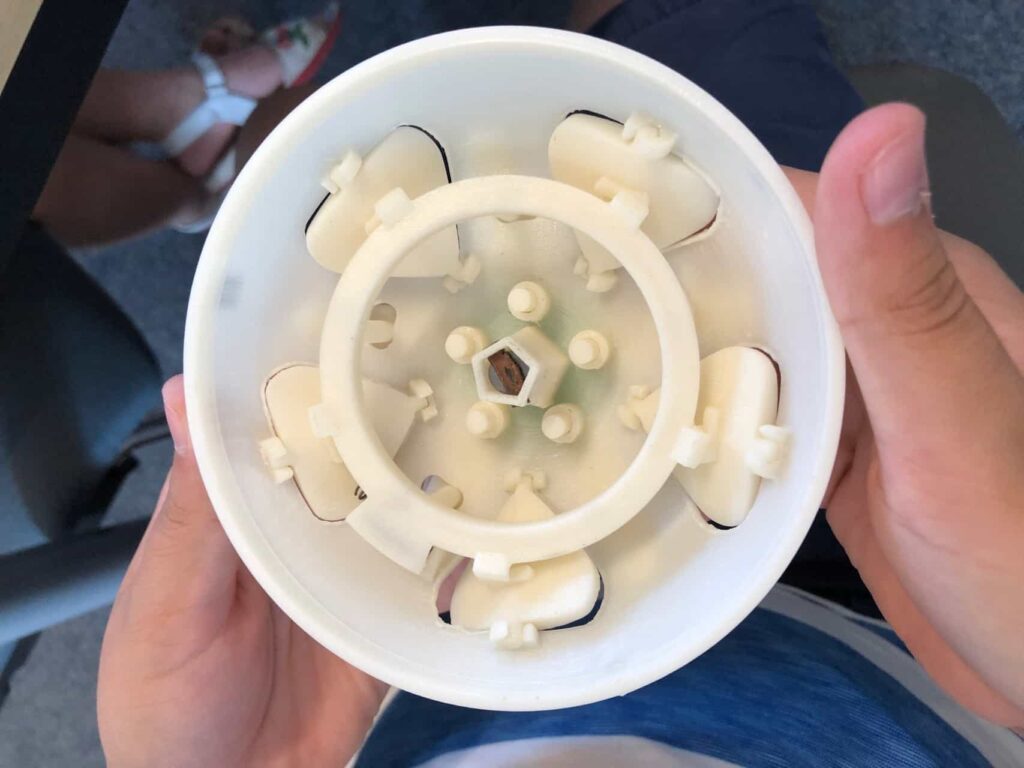
Based on the user testing and feedback, the prototype mechanisms were revised and developed into the final design. The development of the geometric structure from an initial sketch of the protein arrangement to interior and exterior design configuration of the model is illustrated below.
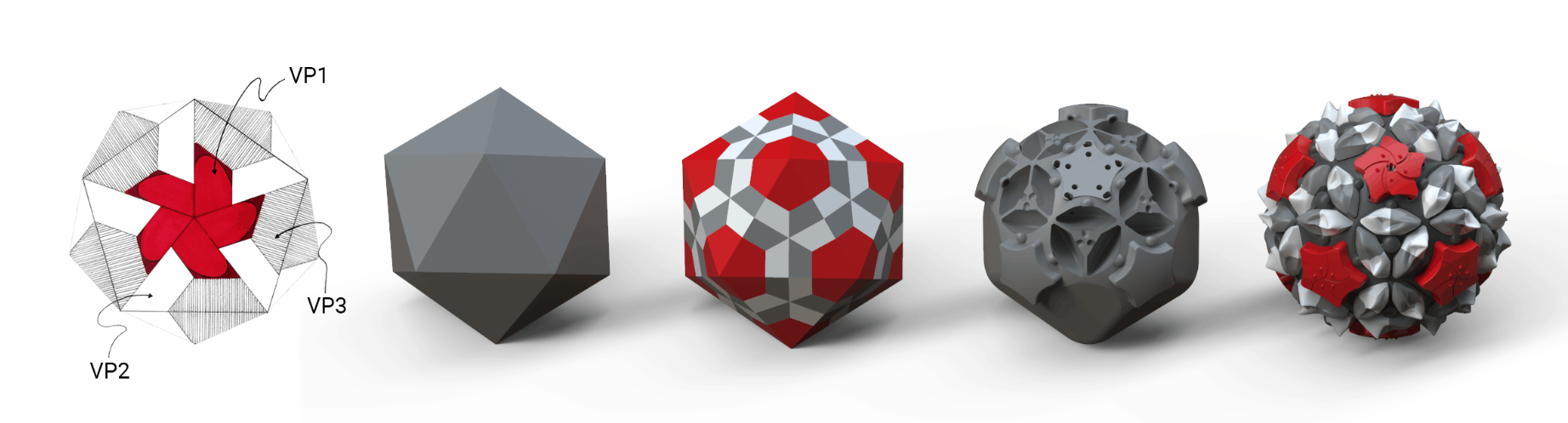
The design of the final prototype, shown below, enables a number of changes to the capsid model as it transforms between states. The widening of the mesa is achieved through the same iris mechanism used in the testing prototype. VP4 proteins, located directly below the top surface of the mesa, slide on a spherical surface and are tied up with an elastic band at one end. This configuration allows the VP4 claw to expand as the mesa opens.
The transformation of the model into its active form also changes the shape of the capsid propellers. The propeller tip, composed of VP2, sinks into the surface while VP3 raises up more prominently. This movement was replicated by making a seesaw-like structure with the pivot point between the proteins. Five of these are arranged around the mesa, with each one connected to the ring, synchronising the parts together.
The internal mechanism of the model is pictured below. To ensure the symmetry of the propellers, the central VP2 and VP3 units are each connected to their counterparts using a triangular piece, which oscillates around the axis of 3-fold symmetry. All of the moving bodies are connected and synchronised, ensuring that movement of one element results in the transformation of the capsid face.
An LED circuit is incorporated to visualise the transmission of genomic material. When the connecting ring is in its inactive position, the circuit remains open. The circuit is closed by the ring turning towards the active position. The battery pack holder and slots for the diode and switch are incorporated into the base frame, reducing the need for additional components.
PAPERS, POSTERS & EXHIBITIONS
Dobras, K., Thomas, B. and Grehan, K. (2020). “Virus Mechanics: Designing a Physical Model for STEAM Learning,” in Bridges Conference Proceedings 2020, pp.379—382.
Dobras, K., Thomas, B. and Grehan, K. (2020). “Virus Mechanics: Designing a 3D Model for Public Engagement with Science,” BCUR 2020.
Dobras, K. and Thomas, B. (2020). “PolioMechanics 1.0,” in Bridges Mathematical Art Gallery (online), 2020.